Enhancing Global Sustainability: The Role of the Blue Economy in Preserving Natural Capital
Abstract:
This investigation delineates the critical role of the Blue Economy in preserving the planet's natural capital, a cornerstone for sustainable development. A systematic analysis of theoretical research and policy documents was conducted to elucidate the integration of economic systems with environmental conservation. Correlation and regression analyses were employed to evaluate the interactions between economic activities and the status of natural capital, with particular emphasis on Gross Domestic Product (GDP), population growth, ecological footprints, and biocapacity over the period from 1994 to 2020. The results revealed that prevalent economic practices are contributing to significant depletion of natural capital, thereby posing severe risks to both ecological and economic stability. Moreover, the efficacy of the Blue Economy in mitigating these risks was demonstrated, showcasing its potential to align economic growth with environmental preservation. This study provides compelling evidence that a transition towards the Blue Economy is not merely viable but imperative for sustainable development. The implications of these findings are pivotal for policymakers, stakeholders, and industries, underscoring the urgent need to revise economic strategies to prioritize environmental sustainability. Such a shift is deemed crucial for realizing long-term sustainability goals and ensuring economic resilience in the face of environmental challenges.1. Introduction
The imperative to transform our existing economic systems is underscored by the pressing challenges posed by climate change, largely attributed to anthropogenic activities. The revision of economic development concepts towards sustainable development has become crucial. Sustainable development rests on three foundational pillars: the conservation of natural capital, economic growth, and the promotion of social welfare. In response to these challenges and to advance the Sustainable Development Goals (SDGs), the concept of the Blue Economy has been introduced and operationalized. It extends the Green Economy's reach and fosters a culture of ecosystem stewardship.
Recent climate trends have brought the urgency of this transformation to the forefront. Data from the Copernicus Climate Change Service highlights that 2023 marked one of the warmest periods on record, signaling an undeniable trend of global warming with significant ramifications for the biosphere (Copernicus, 2024). The National Aeronautics and Space Administration (NASA) has indicated that the oceans, which absorb 90% of this increased heat, are central to understanding global warming's impacts (NASA Sea Level Change Portal, 2024). The year 2023 also witnessed substantial human and economic losses due to natural phenomena, exacerbating the need for effective climate adaptation and mitigation strategies. In 2022 alone, natural phenomena were responsible for more than 70,000 deaths, with over 60,000 attributed to extreme temperatures (Our World in Data, 2022). Such an increase in the Earth's surface temperature affects the hydrological cycle and disrupts water balance, leading to climate change with profound socio-economic consequences. In 2022, the economic losses from natural disasters amounted to 313 billion USD, significantly exceeding the anticipated 132 billion USD.
These environmental shifts prompt destructive weather events, diminish freshwater availability, and challenge food security. Additionally, the rise in sea levels and increased soil degradation are direct consequences of the warming climate, affecting human and ecological systems profoundly. Climate change is catastrophic for humanity, but it is caused by human activities that destroy the natural capital of the planet (Ehrlich & Holdren, 1971; Pincetl, 2015). In order to slow down the process of destruction of the biosphere, it is important to protect it from anthropogenic influence, change the existing approach to the economy, and develop mitigating and adaptation activities. At the same time, adaptation should be aimed at protecting and preserving natural resources from the influence of human activity.
In this context, protecting the biosphere from anthropogenic harm and reorienting economic frameworks toward sustainability are paramount. Addressing biocapacity and the ecological footprint offers a lens to assess and respond to ecological deficits at various scales. This study is structured to explore the Blue Economy concept, assess its understanding and application across different sectors, and investigate its integration into macroeconomic science. Through this analysis, the research aims to illuminate the interplay between economic systems and environmental integrity, offering insights into sustainable economic transformations that prioritize the planet's natural capital. This study addresses several research questions that guide our investigation into the relationship between economic systems and environmental sustainability:
RQ1. Investigate how the Blue Economy is conceptualized and applied within academic and non-academic contexts, determining if this understanding aligns with sustainable development principles that emphasize the preservation of resources for future generations.
RQ2. Study the incorporation of Blue Economy principles in macroeconomic science, particularly in addressing economic sustainability amidst the planet's limited and vulnerable natural resources.
RQ3. Examine the socio-economic impacts of failing to preserve and restore natural capital, focusing on the critical relationship between the biosphere's health and socio-economic well-being.
This article draws on a rich background of research in ecological economics and the greening of macroeconomic models. Central to this discussion is Daly (2015), who articulated the consequences of linear economic growth and emphasized the integration of ecological concerns into macroeconomic science, portraying the economic system as a subsystem of the biosphere. Heyes (2000) furthered this integration by introducing the concept of an Ecological Equilibrium (EE) curve into macroeconomic models. Lawn (2003) expanded on Heyes' ideas, discussing the drivers and implications of shifts in this EE curve.
Significant attention has also been paid to the socio-economic impacts of climate change. Khurshid et al. (2022) and Kalkuhl & Wenz (2020) analyzed the socio-economic consequences and costs associated with carbon emissions and non-market losses from extreme weather events and sea-level rise, assessing their broader impact on economic activity. Morales (2007) explored environmental policies within macroeconomic frameworks, advocating for economics to support sustainable and human development goals.
Tol (2009) examined the economic losses attributable to climate change, including biodiversity loss and violent conflicts, and discussed the varying impacts on countries with different income levels while emphasizing the social value of carbon emissions. Irfan & Alatawi (2019) explored the synergistic relationships between biosphere components, highlighting the critical role of aquatic ecosystems in sustaining human life and promoting well-being in the face of environmental threats. Lloyd & Shepherd (2020) discussed how climate change could precipitate ecological catastrophes affecting both human and natural systems. Lamberti et al. (2010) underscored the necessity of developing interdisciplinary approaches to address the vulnerability of aquatic ecosystems to climate change and anthropogenic activities. Recent studies have focused on the well-being dimensions associated with the Blue Economy, cautioning against prioritizing material aspects at the expense of the subjective benefits derived from sustainable economic development (Fudge et al., 2023). Malhi et al. (2020) investigated the interplay between climate change and the biosphere, particularly the vulnerability of ecosystems and their role in enhancing resilience to climate impacts.
In addition to scholarly literature, this research examined documents from non-academic organizations and institutions that influence socio-economic policy and specialize in the study of natural resources, such as the European Commission, the Organization for Economic Cooperation and Development (OECD), the United Nations (UN), the United Nations Environment Program (UNEP), and the Commonwealth of Nations. The study compared these practical policy approaches with the theoretical perspectives that view the economy as a subsystem of the biosphere, providing a comprehensive overview of the challenges and strategies involved in integrating ecological concerns into economic policies.
This study explores the interconnections between economic systems and the biosphere's subsystems, which are conceptualized as natural capital and the foundational elements supporting the existence of the economic system. The analysis traces the evolution of the mainstream economic paradigm, particularly its gradual separation of environmental issues from the core concepts of economic development. Additionally, this paper introduces a graphical model that underscores the critical importance of developing the economic system's protective functions in relation to the planet's natural capital.
As a result of our investigation, we synthesized views from various scientists on the consequences of neglecting the protection and conservation of natural capital. This synthesis is visually represented in a comprehensive graphical model that delineates the boundaries of economic growth in relation to ecological limits. Based on our findings, we advocate for a significant transformation of the existing economic system through the adoption of the Blue Economy concept. This concept fosters a holistic perception of the economic system as deeply intertwined with natural capital, promoting a sustainable integration of environmental and economic objectives.
This research provides valuable insights for stakeholders looking for alternatives to the conventional focus on the physical protection and conservation of the planet's natural capital. The findings and methodologies employed herein are applicable not only within the economic sphere, but also in related disciplines that focus on the preservation and protection of ecosystems, viewing them as cohesive structures with synergistic interdependencies.
2. Extended Background
The evolution of society is inherently linked to economic growth, which, within the traditional economic paradigm, is constrained by limited resources. As demand for goods and services increases, so too does their value. Although technological advancements improve the efficiency of resource use and meet long-term consumer needs, they paradoxically exacerbate the limitations of resources. This phenomenon, known as Jevons' Paradox (Alcott, 2005), illustrates how technological progress, while conserving resources in some areas, leads to their overall depletion.
This trend is consistent across all inputs, reinforcing the mainstream view that economic activity primarily revolves around the circulation of resources, supplied predominantly by households. These inputs, including natural capital, are consumed without restrictions on resource inputs or emissions. Yet, significant changes like the cyclical temperature variations linked to natural phenomena such as La Niña and El Niño are primarily driven by human activities. These activities, intentional or otherwise (Gill & Malamud, 2017), result in notable anthropogenic impacts on the Earth's climate, as evidenced by increased heat emissions (Zhao et al., 2014).
The degradation of natural capital, accelerated by climate change, is further intensified by transportation, industrial emissions, mining, incineration, and even the environmental impacts of warfare. In response, international agreements like the Paris Agreement have been established to curb global warming by limiting harmful emissions.
The concept of the "Blue Economy" has emerged as an extension of the "Green Economy," focusing on the sustainable use of oceanic and coastal resources. It aims to improve human well-being and social justice while reducing environmental risks and ecological scarcity. This shift was catalysed by the intensification of climate-related disasters, prompting a re-evaluation of fiscal policies towards more direct protection of natural capital and emphasizing adaptation strategies as crucial for the sustainable development of the "Blue Economy".
Historically, significant shifts in environmental policies have been marked by a series of UN conferences, notably the United Nations Conference on Human Environmental Problems in 1972, which led to the establishment of the United Nations Environment Program (UNEP). More recent discussions during the 2012 United Nations Conference on Sustainable Development, "Rio+20", have reinforced the importance of integrating low carbon, resource-efficient, and socially inclusive principles into the economic systems of developing coastal nations that heavily depend on marine resources.
Gunter Pauli introduced the "Blue Economy" to the scientific community in 2009, advocating for an economic model that transcends mere conservation to focus on the regeneration of ecosystems ("Blue Economy: 10 years, 100 innovations, 100 million jobs", Club of Rome). This has given rise to two parallel narratives within the "Blue Economy": one that aligns closely with the "Green Economy," utilizing market mechanisms to protect nature, and another that emphasizes a culture of regeneration and sustainable adaptation of ecosystems.
Therefore, it is fair to consider the "Blue Economy" as a policy (Kwiatkowski & Zaucha, 2023), in the context of expanding the sphere of influence of the "Green Economy", on the one hand, and culture, which forms a fundamentally different attitude toward the world, requires a qualitative transformation of the economic system, on the other hand (Fudge et al., 2023; Okafor-Yarwood et al., 2020).
In 2021, the European Commission communicated a new sustainable Blue Economy framework for the EU, emphasizing the transformation of the economic landscape to ensure it is modern, competitive, and capable of preserving the EU’s natural capital. This initiative aligns with the goals of the European Green Deal, aiming to propel actions and ideas that foster a sustainable economic future.
At its core, the Blue Economy is committed to the sustainable use of oceanic, coastal, and sea resources. As research progresses, it reveals the complex, sometimes paradoxical relationships between various elements of the biosphere and hydrosphere (Zhao et al., 2021), highlighting the challenges and opportunities in navigating the green transition and blue transformation toward sustainability.
Herman Daly posits that the economy is not an isolated system but rather a subsystem of the biosphere; thus, its condition directly impacts economic stability (O’Neill, 2022). Given that the biosphere encompasses the hydrosphere, atmosphere, and lithosphere (Kabata-Pendias & Mukherjee, 2007), it is essential to consider the economic system within the synergistic relationships among these subsystems. These relationships span various environmental interfaces, including land-air, air-water, and water-land interactions (Suni et al., 2015). Moving forward, our research will explore the interactions between the economic system and nature comprehensively, considering the biosphere as an interconnected whole rather than isolating its individual elements.
Daly (2015) also argues that economic growth often entails ecological sacrifices. In a scenario he describes, economic growth diminishes ecosystem services that are abundant in a less populated, "empty" world but become scarce in a densely populated, "full" world. As ecosystem services diminish, the costs associated with them decrease at an increasing rate, leading to the displacement of the ecosystem by economic activities. Initially, this displacement is recognized as economic growth, beneficial up to a certain limit. Beyond this economic limit, growth becomes "uneconomic" as it starts costing more than it benefits, thus impoverishing society rather than enriching it. Continuing on this path of unbounded growth will inevitably push the economy to the brink of disaster, challenging the sustainability of both natural resources and the overall economic system (Figure 1).

This figure illustrates Herman Daly's model of limits to growth, highlighting three critical thresholds that define the sustainability of economic expansion: the limits of futility, ecological disaster, and economic boundaries. Each threshold represents a significant point in the relationship between economic activities and ecological capacity:
· Limits of Futility: This limit is reached when the marginal utility of production drops to zero. At this point, even if production costs are nil and GDP continues to increase, the potential for consumption and the benefits derived from it are capped, leaving welfare indicators stagnant.
· Limits of Ecological Disaster: Depicted as a sharp upward pivot in the marginal cost curve to a vertical orientation, this limit represents a critical juncture where the exact nature of an environmental shock can drastically change the economy’s trajectory. Climate change is commonly recognized as a primary catalyst for reaching this disaster threshold.
· Economic Limits: This threshold occurs where marginal costs meet marginal benefits, resulting in optimized net benefits. It typically precedes the futility limit but may overlap with the disaster limit in severe scenarios.

The figure visually encapsulates these concepts, demonstrating the progression from beneficial growth to potential detriment if ecological limits are ignored, thereby emphasizing the importance of integrating sustainable practices within economic planning to avert critical ecological and economic crises. In response to Daly’s critique of the traditional Hicks-Hansen macroeconomic model (IS-LM model) for failing to realistically incorporate ecological constraints (Daly, 1991; Durlauf & Hester, 2008), Anthony Heyes adapted this model (Figure 2) to better meet the demands of ecological economics by introducing the EE curve (Heyes, 2000). The EE equilibrium is achieved when the rate of resource use aligns with the natural recovery rate of the environment (the boundaries of economic and non-economic growth in the empty and full worlds are divided by color, respectively).
Integrating the EE curve into traditional economic models demonstrates that addressing environmental issues requires a more comprehensive approach than micro-level considerations alone. As Jaffe et al. (2005) and Lawn (2003) suggest, macroeconomic frameworks must expand to include ecological variables to accurately reflect the interactions between economic activities and environmental impacts.
Morales (2007) further advances this discourse by arguing that the greening of the economy should transcend mere policy adjustments and become a foundational economic principle. This shift would ensure that sustainability is not just an incidental effect but a central objective of economic policymaking. By systematically incorporating environmental sustainability into the macroeconomic models, such as by extending the IS-LM framework to include the EE curve, economies can develop mechanisms that genuinely support long-term ecological balance and economic health. The EE curve is characterized by a downward trajectory but is not a linear function of the long-run interest rate (R). According to Morales (2007), as R decreases, investments in recovery technologies increase. However, once the potential for technological improvements is exhausted, further reductions in R no longer stimulate additional investment; this results in the EE curve becoming vertical as R approaches zero.
Lawn (2003) expanded the Heyes model to incorporate the dynamics of pollution permits and technological progress. He introduced two parameters: β, an institutional parameter that quantifies the extent to which the costs associated with resource depletion and pollution are borne by the user and polluter (0 ≤ β ≤ 1), and γ, a technological parameter that reflects the level of resource conservation and the effectiveness of pollution-reducing technology (0 ≤ γ ≤ 1). Lawn's adjustments demonstrate how shifts in the ecological balance curve can occur and posit that these shifts allow for the expansion of the economic system while managing ecological impacts.
Further elaborating on this model, Das et al. (2023) highlight the practical applications and implications of these parameters in current economic policies, illustrating the dynamic interplay between economic growth, environmental sustainability, and technological innovation (Figure 3).
Over time, the limitations of scientific and technological progress become apparent in their ability to effectively protect natural capital. Investments in environmental protection activities, even with minimal interest rates, will become costlier than the potential profits they generate, causing production to plateau. This is represented by the vertical segment of the EE curve, at which point the curve becomes completely inelastic. Should the EE curve shift to the right of the macroeconomic equilibrium, it indicates that the economy still has potential for growth. The institutional parameter β and the technological parameter γ play critical roles in maintaining or stimulating this rightward shift of the curve, ensuring economic growth does not compromise ecological stability.


Lhote (2022) adds that environmental shocks can cause the EE curve to shift either leftward or rightward relative to the macroeconomic equilibrium point. A leftward shift occurs due to negative environmental shocks or a decrease in the availability of natural capital, potentially reducing it to zero. This dynamic aligns with Herman Daly's model of economic growth, emphasizing the concept of an environmental limit to economic expansion, beyond which lies the risk of ecological catastrophe (Figure 4).
Herman Daly highlights an ideal model where macroeconomic equilibrium aligns with biophysical equilibrium. He critiques the post-World War II economic boom, which led to what he terms an 'empty world'—a scenario marked by rapid growth and perceived endless resources. Daly contrasts this with today's 'full world,' where natural capital, such as fish populations, is the limiting factor, not the mere capacity for human activity, such as the number of fishing vessels. He notes that increasing the number of boats no longer correlates with an increase in fish caught, as the true limit is now the reproductive capacity of the fish populations themselves (Daly, 2015).
In this context, the 'limit of ecological disaster' is represented by a vertical shift in the EE curve, occurring when institutional and technological advancements (represented by β=1 and γ=1) are maximized. Beyond this point, any additional economic activity becomes a negative shock, driving the EE curve towards zero production (Y=0) and signaling the depletion of natural capital.
Figure 5 visualizes a combined model that correlates the concept of economic growth with ecological thresholds. It depicts how unchecked growth eventually leads to an ecological disaster, marked as the point of central symmetry in the model. This critical point, or ecological limit, results from neglecting the planet's natural capital.

The diagram clearly illustrates the consequence of reaching this limit: the regeneration of natural capital becomes untenable. A negative shock to the ecological system is represented, causing the ecological balance curve to shift progressively toward zero, indicating complete depletion of natural capital. This model serves as a graphic warning of the severe repercussions of surpassing ecological boundaries in the pursuit of economic expansion.
3. Data and Research Methodology
To address these research questions, the study was conducted in three distinct stages. In the first stage, an extensive review of scientific literature and working papers from non-academic institutions was undertaken to explore shifts in the mainstream economy. This analysis was particularly focused on how environmental considerations are integrated, how natural capital is delineated, and how the concept of Blue Economy is being developed.
In the second stage, a theoretical approach was employed to systematically analyse and organize scientific articles that incorporate environmental issues into macroeconomic theory. This helped in tracing the evolution of economic thought in this area. Building on existing models that merge economic development with environmental concerns, a comprehensive model was developed. This model theoretically illustrates the consequences of economic growth and the planet’s natural capital under the scenario of linear resource use and adherence to mainstream economic narratives.
The third stage involved performing correlation and regression analyses to establish and quantify the strength of the relationships between the economic system and the processes affecting the biosphere. This quantitative analysis aimed to provide empirical support for the theoretical model (Growth Limits to Ecological Limits) developed in the previous stage.
To confirm the existence and assess the strength of the relationship between the economic system and natural capital, a statistical analysis of online secondary data for the period 1994–2020 from the OECD, the World Bank, the Ecological Footprint Network, and FAO databases (FAO, 2024; OECD, 2024; The Ecological Footprint Network, 2024; The World Bank, 2024) was conducted and processed using the add-on Data Analysis in Microsoft Excel using correlation and regression methods. The data collection process was conducted to find variables that would characterize the state of the economy (GDP, population, greenhouse gas emissions), the state of the biosphere (mean temperature change of the meteorological year, total biocapacity and biocapacity per person, total ecological footprint, and ecological footprint per person), as well as data on environmental tax revenues and fees for the use of intellectual property, as they can act as a deterrent and potentially reduce or control impacts on natural resources.
A p-value threshold of 0.05 was applied to determine statistical significance for correlation results. A p-value greater than 0.05 indicates that the relationship between the variables is not statistically significant, while a p-value less than 0.05 indicates a significant effect of one variable on the other.
Correlation analysis showed the existence of a relationship between variables at the level of 0.227–0.996 in absolute value. The analysis confirmed the existence of relationships between the studied variables, where 84.4% of the variables demonstrated a very strong relationship (Pearson r ≥ 0.7), 3.3% - a strong relationship (Pearson r: 0.5–0, 69), and 2.2% – weak connection (Pearson r: 0.1–0.29).
For regression analysis, six dependent variables were chosen: changes in the temperature of the planet; greenhouse gas emissions; total biocapacity and biocapacity per person; total ecological footprint and ecological footprint per person, for each of which a set of predictors was proposed, forming 34 pairs. In some cases, dependent variables became predictors. For example, if change in temperature is the dependent variable, total biocapacity is the predictor, and in the case where total biocapacity is the dependent variable, change in temperature is the predictor. Due to the presence of signs of collinearity between the variables revealed as a result of the correlation analysis, a simple linear regression is used for the regression analysis, the purpose of which is to assess the influence of the independent variable on the dependent one, which is determined through regression coefficients (beta values). Only one of the 34 pairs was found to have a p value greater than 0.05, indicating no statistical significance for that pair. In addition, 58.8% of the pairs analyzed had beta coefficients ranging from 2.78E-15 to 9.84E-05. Given these findings, we conclude that, while the predictors generally have very weak effects on the variables, any observed shifts in the dependent variables are primarily due to changes in the predictors.
These methodological steps ensured a robust analysis, providing a solid empirical foundation to the theoretical insights developed earlier in the study. The findings from this comprehensive approach not only validate the theoretical models proposed but also offer practical insights for policymakers and stakeholders engaged in shaping sustainable economic practices.
4. Results and Discussion
This section of our analysis delves into the interconnections between economic growth (measured by GDP), demographic trends (population growth), and their environmental impacts, particularly through greenhouse gas emissions. Our findings indicate that as GDP and population increase, so do the emissions of greenhouse gases, leading to an expanded ecological footprint. This exacerbates the imbalance between the ecological footprint and biocapacity, highlighting a growing discrepancy between resource use and natural resource regeneration capabilities. In addition, in this section, we verify whether environmental tax revenues and fees for the use of intellectual property really have an impact on the state of the planet's natural capital, as they can act as deterrents and potentially reduce or control impacts on natural resources.
Table 1 presents the descriptive statistics for key indicators analysed over the period from 1994 to 2020. It encapsulates trends and variations in economic growth, population changes, greenhouse gas emissions, and other factors influencing natural capital and ecological footprints.
Notably, the total ecological footprint has increased at a faster rate than the growth of total biocapacity, indicating an escalating scarcity of natural resources. Per capita analysis, detailed in Appendix 1, reveals a consistent downward trend in biocapacity per person throughout the study period, in contrast to the ecological footprint, which shows a different pattern. The overall changes in these variables are predominantly driven by variations in temperature and greenhouse gas emissions, as further explored in Table 2 and Table 3.
These findings highlight the urgent need for strategies that address the growing imbalance between ecological footprint and biocapacity, focusing on sustainable resource management and environmental conservation to mitigate the adverse effects of these trends.
Unit of Measure | N | Mean | Median | Minimum | Maximum | |
Mean temperature | Change of meteorological year | 27 | 1.03 | 1.00 | 0.40 | 1.70 |
Total greenhouse gas emissions | kt of CO2 equivalent | 27 | 40,140,764 | 41,308,022 | 31,131,196 | 48,089,617 |
Total biocapacity | GHA | 27 | 11,625,717,057 | 11,573,652,916 | 11,124,642,099 | 12,045,506,800 |
Biocapacity | GHA per person | 27 | 1.74 | 1.72 | 1.53 | 1.97 |
Total ecological footprint | GHA | 27 | 17,815,651,067 | 18,181,300,455 | 14,096,093,639 | 20,599,120,928 |
Ecological footprint | GHA per person | 27 | 2.64 | 2.64 | 2.47 | 2.80 |
GDP | USD | 27 | 56,421,735,993,733 | 58,446,784,384,979 | 279,975,060,90,383 | 87,777,403,956,321 |
Population | total | 27 | 6,727,960,467 | 6,717,567,584 | 5,642,046,034 | 7,820,205,606 |
Charges for the use of intellectual property, payments | USD | 27 | 221,941,015,582 | 210,208,347,079 | 30,901,646,730 | 462,327,512,886 |
Environmentally related tax revenue | USD | 27 | 2,140,146 | 2,360,913 | 1,346,120 | 2,741,532 |
1. Mean Temperature | 2. Total Greenhouse Gas Emissions | 3. Total Biocapacity | 4. Biocapacity per Person | 5. Total Ecological Footprint | 6. Ecological Footprint per Person | 7. GDP | 8. Population | 9. Charges for the Use of Intellectual Property | 10. Environmentally Related Tax Revenue | |
Var. 1 | 1 | |||||||||
p-value | Effect size interpretation | |||||||||
Var. 2 | 0.801 | 1 | Ignored | 0.01-0.9 | ||||||
p-value | 0.000 | Low | 0.1-0.29 | |||||||
Var. 3 | 0.826 | 0.965 | 1 | Moderate | 0.3-0.49 | |||||
p-value | 0.000 | 0.000 | Strong | 0.5-0.69 | ||||||
Var. 4 | -0.858 | -0.984 | -0.975 | 1 | Very strong | ≥ 0.7 | ||||
p-value | 0.000 | 0.000 | 0.000 | |||||||
Var. 5 | 0.756 | 0.990 | 0.951 | -0.971 | 1 | |||||
p-value | 0.000 | 0.000 | 0.000 | 0.000 | ||||||
Var. 6 | 0.227 | 0.641 | 0.510 | -0.554 | 0.726 | 1 | ||||
p-value | 0.000 | 0.000 | 0.000 | 0.000 | 0.000 | |||||
Var. 7 | 0.785 | 0.991 | 0.962 | -0.972 | 0.970 | 0.584 | 1 | |||
p-value | 0.000 | 0.000 | 0.000 | 0.000 | 0.000 | 0.000 | ||||
Var. 8 | 0.861 | 0.981 | 0.986 | -0.996 | 0.960 | 0.507 | 0.977 | 1 | ||
p-value | 0.000 | 0.000 | 0.000 | 0.000 | 0.000 | 0.000 | 0.000 | |||
Var. 9 | 0.831 | 0.979 | 0.975 | -0.976 | 0.949 | 0.496 | 0.989 | 0.987 | 1 | |
p-value | 0.000 | 0.000 | 0.000 | 0.000 | 0.000 | 0.000 | 0.000 | 0.000 | ||
Var. 10 | 0.718 | 0.979 | 0.914 | -0.943 | 0.981 | 0.738 | 0.967 | 0.930 | 0.932 | 1 |
p-value | 0.0000 | 0.000 | 0.000 | 0.000 | 0.000 | 0.000 | 0.000 | 0.000 | 0.000 |
Response Variables | Predictor Variables | β-coefficient | p-value |
Mean temperature | Total greenhouse gas emissions | 4.32E-08 | 0.000 |
GDP | 1.16E-14 | 0,000 | |
Population | 4.07E-10 | 0.000 | |
Charges for the use of intellectual property | 1.77E-12 | 0.000 | |
Environmentally related tax revenue | 4.45E-07 | 0.000 | |
Total biocapacity | 9.43E-10 | 0.000 | |
Total greenhouse gas emissions | Population | 0.009 | 0.000 |
GDP | 2.71E-07 | 0.000 | |
Charges for the use of intellectual property | 3.85E-05 | 0.000 | |
Environmentally related tax revenue | 11.238 | 0.000 | |
Total biocapacity | Mean Temperature change | 724,144,448 | 0.000 |
Total greenhouse gas emissions | 45.688 | 0.000 | |
GDP | 1.25E-05 | 0.000 | |
Population, total | 0.409 | 0.000 | |
Charges for the use of intellectual property | 0.002 | 0.000 | |
Environmentally related tax revenue | 496.519 | 0.000 | |
Total ecological footprint | Mean temperature | 5,194,407,881,110 | 0.000 |
Total greenhouse gas emissions | 367.403 | 0.000 | |
GDP | 9.84E-05 | 0.000 | |
Population | 3.124 | 0.000 | |
Charges for the use of intellectual property | 0.014 | 0.000 | |
Environmentally related tax revenue | 4 176/193 | 0.000 | |
Total biocapacity per person | Mean temperature | -0.3637 | 0.000 |
Total greenhouse gas emissions | -2.25E-08 | 0.000 | |
GDP | -6.08E-15 | 0.000 | |
Population | -2.00E-10 | 0.000 | |
Charges for the use of intellectual property | -8.79E-13 | 0.000 | |
Environmentally related tax revenue | -2.48E-07 | 0.000 | |
Total ecological footprint per person | Mean temperature | 7.29E-02 | 0.256 |
Total greenhouse gas emissions | 1.11E-08 | 0.000 | |
GDP | 2.78E-15 | 0.001 | |
Population | 7.73E-11 | 0.007 | |
Charges for the use of intellectual property | 3.39E-13 | 0.009 | |
Environmentally related tax revenue | 1.47E-07 | 0.000 |
Table 2 displays the correlation coefficients between various economic indicators and the state of natural capital over the study period. It includes variables such as GDP, population growth, greenhouse gas emissions, and ecological footprint measures. These correlations help elucidate the extent to which these economic activities are linked to changes in natural capital, providing crucial insights into their interdependencies and the impact of economic activities on environmental health.
Key findings from the table show that with a 0.1-unit increase in temperature, total biocapacity increases by 724 million GHA, whereas the total ecological footprint increases by 5,194 million GHA, which is 7.2 times faster. Additionally, an increase in greenhouse gas emissions per unit is associated with an eightfold larger increase in the total ecological footprint compared to the increase in total biocapacity. In contrast, a one percentage point increase in the use of renewable energy has been demonstrated to reduce CO2 emissions per capita by 1.25% (Szetela et al., 2022). These data suggest that mitigating the catastrophic environmental changes associated with population growth, consumption, and production may require the adoption and implementation of economic activities that focus on the sustainable use and preservation of natural resources.
Moreover, the roles of taxation and charges for the use of intellectual property, which could serve as mechanisms to alleviate pressure on natural capital, appear limited mainly to generating revenue rather than actively contributing to resource conservation. These mechanisms predominantly act as payments for the use of natural resources and facilitate their incorporation into production processes that support economic growth, underscoring the need for more direct and effective environmental policies.
Table 3 presents the β-coefficients, which quantify the influence of various economic indicators on the state of natural capital. It provides a comprehensive analysis of the relationships between key variables such as GDP, population growth, greenhouse gas emissions, and their impacts on ecological metrics like biocapacity and the ecological footprint. These β-coefficients are essential for identifying the strength and significance of these relationships, offering invaluable insights for formulating targeted environmental and economic policies.
Our study's backbone, founded on ecological economics and macroeconomic models that integrate environmental variables, sets a robust stage for examining the theoretical implications of economic activities on the natural environment. In our literature review, we explored the concept of ecological limits as proposed by Daly (2015), which posits that unchecked economic growth can lead to significant ecological and economic instabilities. Building on this, Heyes (2000), Lawn (2003), and Lhote (2022) further developed models that integrate ecological considerations into traditional economic frameworks, thereby enhancing our understanding of the interplay between economic systems and environmental sustainability.
Heyes (2000) adapted the IS-LM model to incorporate ecological factors, while Lawn (2003) developed the EE curve, which introduces environmental limits within macroeconomic models, provide a theoretical foundation for examining the dynamic relationships our empirical data have revealed. Lhote (2022) further elaborates on the impact of environmental shocks on economic equilibriums, emphasizing the precarious balance maintained within these systems. Our empirical findings from the regression and correlation analyses substantiate these theories by demonstrating strong relationships between economic activities (e.g., GDP growth, population increases) and environmental impacts (e.g., changes in biocapacity and ecological footprint metrics). Specifically, the data showing a direct correlation between increased GDP and a higher ecological footprint align closely with Daly’s theoretical assertions that economic expansion often comes at a steep environmental cost.
The comprehensive model we developed in the second phase of our study theoretically illustrates the consequences of economic growth on the planet's natural capital under scenarios of linear resource usage. This model is empirically supported by our findings, where correlation coefficients indicate varied but substantial relationships. For instance, the very strong relationships found between greenhouse gas emissions and ecological footprint indices reflect theoretical discussions on the environmental repercussions of economic systems.
By linking these empirical results back to the theoretical frameworks, our study not only validates but also quantifies the impacts discussed in earlier economic-environmental theories. It highlights the practical implications of such theoretical models when applied to real-world data, offering a nuanced understanding of the dynamic interplay between economic activities and ecological health.
Moreover, the discussion reiterates the necessity of adopting economic policies that integrate environmental sustainability at their core, which echoes the theoretical perspectives advocating for a systemic shift towards economic systems that inherently prioritize ecological considerations, such as those embodied in the Blue Economy concept.
For future research, it is suggested that this intersection of economic and environmental dynamics be further explored through more detailed longitudinal studies that can track changes over time, and additional case studies that provide deeper insights into the effectiveness of specific economic policies and their environmental outcomes. This would enrich the discourse on sustainable economic development by providing concrete examples of how theoretical models can be implemented and evaluated in diverse real-world settings.
Following our analysis, we argue in favor of systemic changes aimed at reducing the pressure on natural systems, given the intricate connections between environmental elements and the socio-economic system. We propose rethinking the approach to economic growth — from a linear to a sustainable model — by embracing environmentally friendly practices in the use of natural resources, pivotal initiatives for the sustainable transformation of the economic system, and further greening of the macroeconomy.
Considering the potential consequences of ecological degradation, it is crucial to transform the existing economic system to expand the throughput system of the planet's natural capital. The need for mitigation and adaptation strategies requires more attention from economic actors and stakeholders. Given that climate change impacts all ecosystems to varying degrees, addressing climate change mitigation and adaptation without disrupting biosphere subsystems is essential (Malhi et al., 2020). This underscores the need to expand and explore physical measures to protect ecosystems, which can be effectively achieved through the development of Blue Economy industries, fostering a culture of ecological respect within the environment.
This section delves into the theoretical frameworks and practical implications of our findings regarding the role of the Blue Economy in sustainable development. Intrinsically linked to oceanic, sea, and coastal activities, the Blue Economy extends the principles of the Green Economy to promote respectful and sustainable interaction with marine ecosystems. The application of the Blue Economy is multifaceted, surpassing traditional economic boundaries to adopt a holistic approach that acknowledges the interconnectedness of the biosphere's elements. It challenges the conventional economic system, which often overlooks the finite nature of natural resources, advocating instead for a model that integrates economic activities with ecosystem health.
Our analysis, illustrated in Figure 1, Figure 2, and Figure 3, contrasts different theoretical perspectives on the exhaustion of natural capital. Figure 1 vividly illustrates the severe consequences of complete natural capital depletion, highlighting the existential threat that unchecked economic growth poses to socio-economic stability through ecological collapse. Figure 2 and Figure 3 introduce the concept of environmental equilibrium into macroeconomic models, suggesting that while natural capital is under strain, it might not be exhaustible if managed with strategic institutional and technological interventions. However, the reliance on seemingly infinite technological progression and institutional remedies as panaceas for environmental degradation is critically evaluated. Despite the potential for technology to mitigate environmental impacts, its limitations become apparent in the narrowing carrying capacity of the biosphere, as depicted in Figure 4. This realization challenges the notion of unlimited natural capital and underscores the urgency of rethinking our economic paradigms.
The integration of growth limitation models with equilibrium models offers a nuanced understanding of how ecological degradation can trigger socio-economic collapse, as depicted in Figure 5. This integrated model demonstrates how the depletion of ecosystems, driven by ecological disasters and climate change, could precipitate a socio-economic downturn.
Our empirical analysis substantiates the theoretical model, showing clear connections between economic activities and the state of natural capital. It reveals the looming scarcity of natural resources if current economic practices persist, underscoring the need for a paradigm shift towards sustainable economic practices.
The discussion confirms the deep and inseparable ties between economic systems and natural capital. It emphasizes the critical need to protect natural capital as part of the broader sustainable development agenda, advocating for the principles of the Blue Economy to foster a culture of respect and stewardship towards the environment. This approach is pivotal in averting the looming ecological crisis, which poses a significant risk to both economic stability and human well-being.
Furthermore, the potential of the Blue Economy to act as a catalyst for cross-sectoral and interdisciplinary collaborations is highlighted. This broader perspective can enrich the application of the Blue Economy, emphasizing holistic and integrated approaches that encompass economic, environmental, and social dimensions. Such collaboration among policymakers, industry stakeholders, scientists, and community leaders is essential to ensuring that the principles of the Blue Economy are effectively integrated into broader economic and environmental strategies.
Moreover, exploring the Blue Economy also involves assessing its scalability and adaptability across different geographical and socio-economic contexts, which is vital for tailoring strategies to local conditions while aligning with global sustainability goals. Additionally, the discussion benefits from a deeper exploration of the challenges and opportunities presented by transitioning to the Blue Economy, including potential trade-offs, policy implications, and the role of innovation in fostering sustainable ocean-based industries.
This study is subject to several limitations that must be acknowledged to ensure a balanced view and guide future research. The data primarily derives from international databases such as the OECD, FAO, the World Bank, and the Ecological Footprint Network. While these sources offer valuable global perspectives, they may not capture localized economic and environmental impacts specific to certain regions, potentially affecting the representativeness of the findings. Particularly, the differences between developing and developed countries might not be sufficiently addressed, which could skew understanding of how the Blue Economy impacts various socio-economic contexts. The reliance on secondary data also introduces potential biases in data accuracy and timeliness, which could influence the robustness of the analyses. Additionally, the regression models used assume linear interactions, which may not fully encapsulate the complex dynamics between ecological and economic systems. Collinearity among variables could further skew the regression outcomes. The study period, covering data from 1994 to 2020, excludes recent developments post-2020, which might present different trends or insights. Moreover, the study focuses extensively on the integration of the Blue Economy within macroeconomic frameworks but less on the socio-cultural dimensions that influence its adoption and effectiveness.
Future research should expand the geographical scope to include regional studies, incorporate primary data to complement secondary sources, develop models that account for non-linear dynamics, update the dataset to include recent global events, and explore the socio-cultural impacts of the Blue Economy, with particular emphasis on differentiating between the needs and capabilities of developing versus developed countries. These steps would provide a deeper understanding of the Blue Economy's role in sustainable development and offer nuanced insights for policymakers and stakeholders.
5. Conclusions
This study underscores a critical narrative: adherence to linear economic models is inevitably leading to the socio-economic system's downfall, driven by the relentless depletion of the biosphere. This stark reality underscores the necessity for a sustainable transformation of the economic system, with a particular emphasis on embracing the principles of the Green and Blue Economies.
While goals such as energy efficiency and reduced carbon emissions are commendable within the framework of sustainable development, their achievement must extend beyond mere reliance on institutional initiatives or technological advancements. Although these strategies enhance the biosphere's resilience and its capacity to meet socio-economic demands, their effectiveness remains inherently limited.
The essence of sustainable development—utilizing resources today without compromising the ability of future generations to meet their own needs—requires a profound shift in our collective mindset. It is crucial to integrate environmental considerations into discussions on development and growth, especially given the stark realities of climate change and its multifaceted impacts on humanity.
By synthesizing diverse scholarly perspectives and integrating established theoretical frameworks, our study presents a comprehensive model that incorporates environmental limitations into economic growth paradigms. This model vividly illustrates the impending exhaustion of the biosphere's capabilities, casting a long shadow over future economic growth and societal well-being, which are intricately linked to ecosystem health.
Consequently, our research advocates for an intensified focus on greening macroeconomic strategies and innovating solutions to alleviate pressures on natural capital. These efforts are crucial not only for their immediate benefits but also for ensuring the long-term prosperity of both economic and human systems, fostering a balanced and thriving future.
Additionally, the role of education and public awareness in promoting a sustainable transformation of the economic system is paramount. Enhancing understanding and support among the general public and decision-makers is essential for a swift and effective transition to practices aligned with the Green and Blue Economies.
Future research should explore the policy frameworks and economic incentives that could accelerate the adoption of Blue Economy principles. Investigating successful case studies where Blue Economy initiatives have yielded tangible environmental, economic, and social benefits will provide valuable lessons and scalable models.
Finally, recognizing the limitations of current strategies is crucial as we confront new environmental challenges and deepen our understanding of ecological systems. Our economic models must evolve to ensure the enduring health and vitality of the planet's ecosystems and the well-being of its inhabitants. This evolution necessitates ongoing innovation and adaptation in our economic practices.
Not applicable.
The authors declare no conflict of interest.
Latin symbols | |
C | consumption |
DU | disutility |
IS | investment-savings |
LM | liquidity preference-money supply |
R | long-run interest rate |
t | time |
U | utility |
Y | real income or GDP in Hicks-Hansen model |
Greek symbols | |
β | hypothetical institutional parameter |
γ | hypothetical technological parameter |
Appendix 1. The dynamics of analyzed data
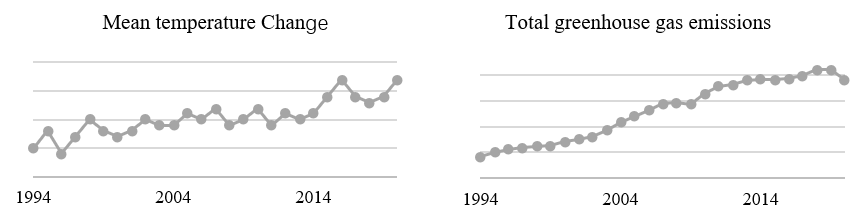
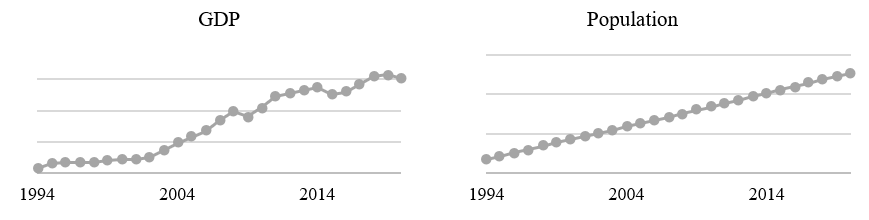
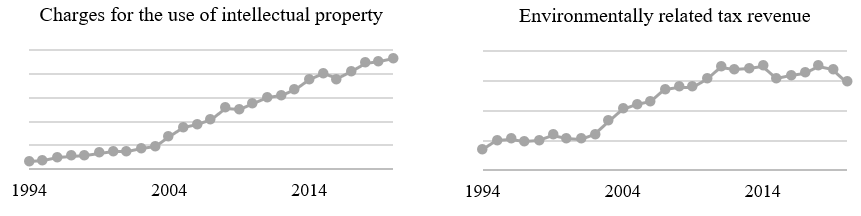
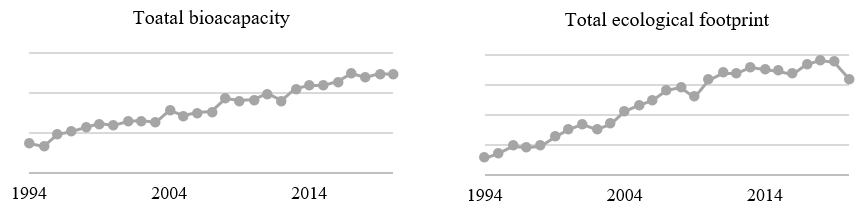
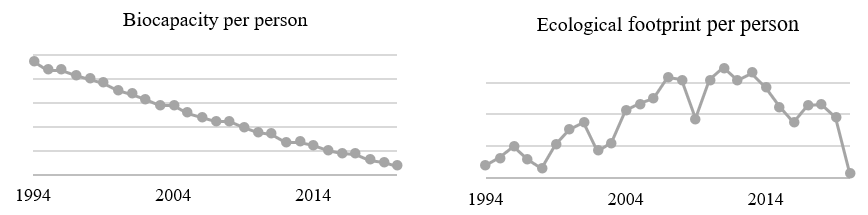
